Abstract
In the pharmaceutical industry, a major challenge is ensuring consistent quality of finished products as the batch scale shifts from laboratory to pilot to commercial levels. This review article aims to provide insights into the current industry practices and understanding of scale-up calculations and factors involved in the production of oral solid dosage forms. Pharmaceutical manufacturing encompasses various unit operations for oral solid dosage forms, including blending, wet granulation, dry granulation via roller compaction, milling, compression, and coating processes such as Wurster and film coating. Each unit operation’s parameters significantly influence the final product’s quality. As batch sizes increase, it becomes crucial to control various process parameters strategically to maintain product consistency. This article discusses the application of scale-up and scale-down calculations throughout different stages of unit operations, highlights the importance of scale-up factors in technology transfer from pilot to commercial scales, and reviews the current methodologies and industry perspectives on scale-up practices.
Keywords
Scale-up, Pharmaceutical industry, Oral solid dosage forms, Granulation, Compression, Film coating
Introduction
Oral solid dosage forms are final drug products designed to be ingested orally. Once swallowed, these forms dissolve in the gastrointestinal tract and the active ingredients are absorbed into the bloodstream. Examples of oral solid dosage forms include powders, granules, tablets, capsules, soft gels, gummies, dispersible films, and pills. These dosage forms are preferred for several reasons: they are relatively easy to administer, they can be clearly distinguished from one another, and their manufacturing processes are well-established and understood. Among oral solid dosage forms, tablets and capsules are the most common. Both consist of an active pharmaceutical ingredient (API), also known as the drug substance, along with various excipients. The manufacturing process for these dosage forms involves several unit operations, including blending, wet granulation (using a rapid mixer granulator or fluid bed processor), dry granulation (via roller compaction), milling, compression, Wurster coating, and film coating [1,2].During the early stages of drug product development, formulations and processes are created using active pharmaceutical ingredients (APIs) and excipients to ensure the quality, safety, and efficacy of the final drug products at the laboratory scale [3]. Once this formulation is established, the process is scaled up from the laboratory to pilot and eventually to commercial scales [4,5]. Throughout this technology transfer, the laboratory-scale formulation is generally finalized and remains unchanged, while process parameters are adjusted. For instance, as the scale of the granulation container increases, both the powder weight and the sizes of components like the impeller and chopper, as well as operational parameters, may need adjustment. These changes can impact the quality of the finished product [6]. Successful scale-up relies on a thorough understanding of the process parameters and the ability to adjust them appropriately to maintain the same quality observed at the laboratory scale.
Successful scale-up of a manufacturing process hinges on a deep understanding of the fundamental principles and insights into each unit operation, which are derived from mechanical insights into the process. The Food and Drug Administration (FDA) has introduced the Quality by Design (QbD) approach to facilitate the efficient and timely production of high-quality pharmaceutical products [7,8]. According to the International Conference on Harmonisation (ICH) guidelines specifically ICH Q8 (Pharmaceutical Development), ICH Q9 (Quality Risk Management), and ICH Q10 (Pharmaceutical Quality System) the scale-up process should be conducted to ensure product quality in alignment with the QbD principles. To meet these regulatory requirements, it is essential to establish methods for reducing variability during scale-up through a systematic understanding of the manufacturing process and the application of the QbD approach [9]. This review examines the application of mathematical considerations in scale-up calculations and explores various methodologies used in scaling up different unit operations for oral solid dosage forms. It aims to provide a systematic strategy to ensure the quality of finished dosage forms in the pharmaceutical industry.
Methods
Scale Up Process Basic Understanding
Using scientific approaches and mathematical calculations for process scale-up or scale-down can significantly reduce the risk of failure, ensure regulatory compliance, and lower costs associated with trial batches. These calculations help to establish robust and realistic parameters for scaling up or down pharmaceutical formulations [10]. When scaling unit process parameters, key considerations include equipment size, shape, working principle, and associated parameters. According to process modeling theory, processes are deemed similar if they exhibit geometric, kinematic, or dynamic similarity.
Scale-Up Strategy for Oral Solid Dosage Forms
The manufacturing of oral solid dosage forms tablets and capsules involves several key unit operations such as blending, granulation, milling, tableting, Wurster coating and film coating. Each of these operations requires a carefully planned scale-up strategy to ensure product quality and process efficiency. Detailed overview of the scale- up strategy for each unit operation are discussed.
A) Blending/Mixing in Pharmaceutical Manufacturing
Blending is a critical unit operation in the manufacture of oral solid dosage forms (e.g., tablets and capsules). It ensures uniformity of the final product by mixing active pharmaceutical ingredients (APIs) with excipients. Equipment used in pharmaceutical blending unit operations are Double-Cone Blenders, Bin Blenders, Octagonal Blenders, V-Blenders and Cubic Blenders [11,12]. The blend should have a degree of homogeneity during blending to ensure the quality of solid dosage forms, such as tablets and capsules [13,14]. The blend homogeneity is influenced by several factors, such as material attributes (for example particle size distribution, particle shape, density, surface properties, particle cohesive strength) and process parameters (for example blender design, rotational speed, occupancy level, and blending time) [15]. These factors affect the agglomeration and segregation of the blend during the blending process, which affect the blend homogeneity. However, experiments with appropriate scale up calculations are sufficient to confirm changes in the agglomeration and segregation of the blend caused by these factors [16]. Scale up considerations and current industry practices in scale up calculations for blending unit operations are presented in Table 1a. Different types of blenders (Figure 1) such as Mass Blenders, Ribbon Blenders, V Cone Blenders, Double Cone Blender, Octagonal Blender, Drum Blender, Bin Blenders and Vertical Blenders, working principles, key factors and advantages are presented in Table 1b.
Table 1a: Process parameters, quality attributes, scale up considerations and industry practices for Blending unit operation.
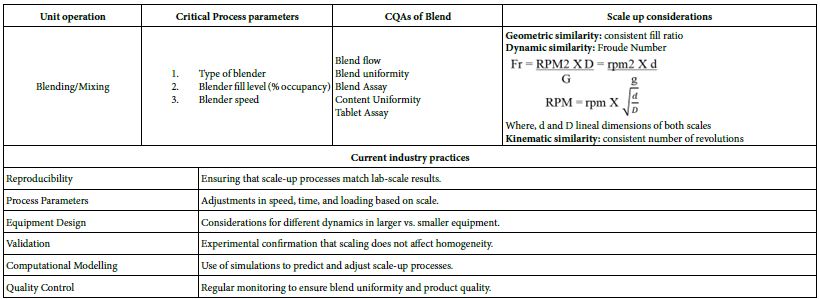
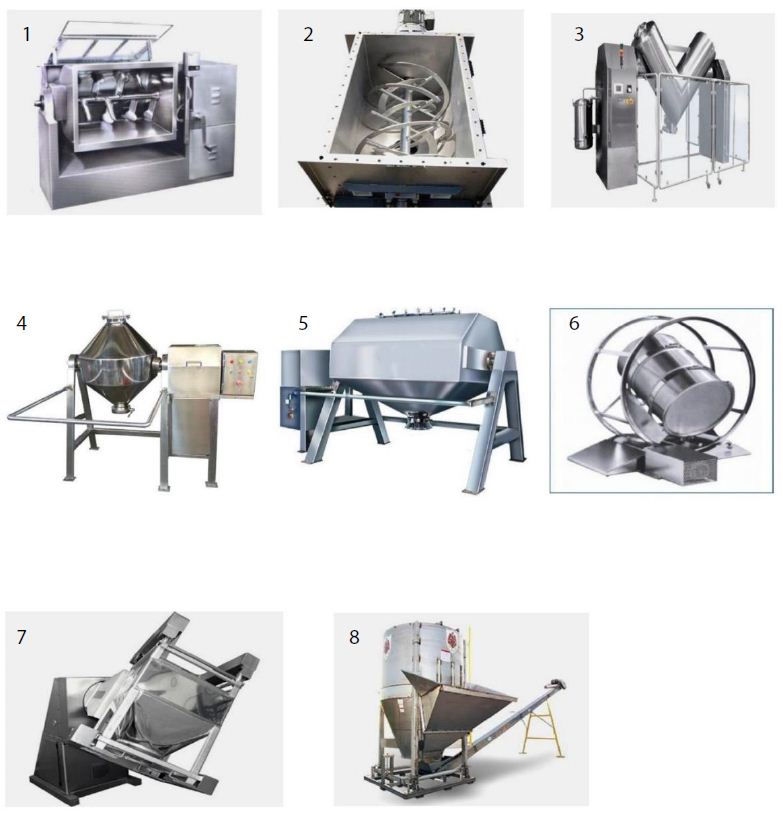
Figure 1: Different types of Pilot/commercial scale model blenders used in pharmaceutical blending unit operation; 1. Mass Blenders; 2. Ribbon Blender; 3. V Cone Blenders; 4. Double Cone Blender; 5. Octagonal Blender; 6. Drum Blender; 7. Bin Blender; 8. Vertical Blender.
Table 1b: Different types of blenders in pharmaceuticals and its working principles, key factors and advantages.
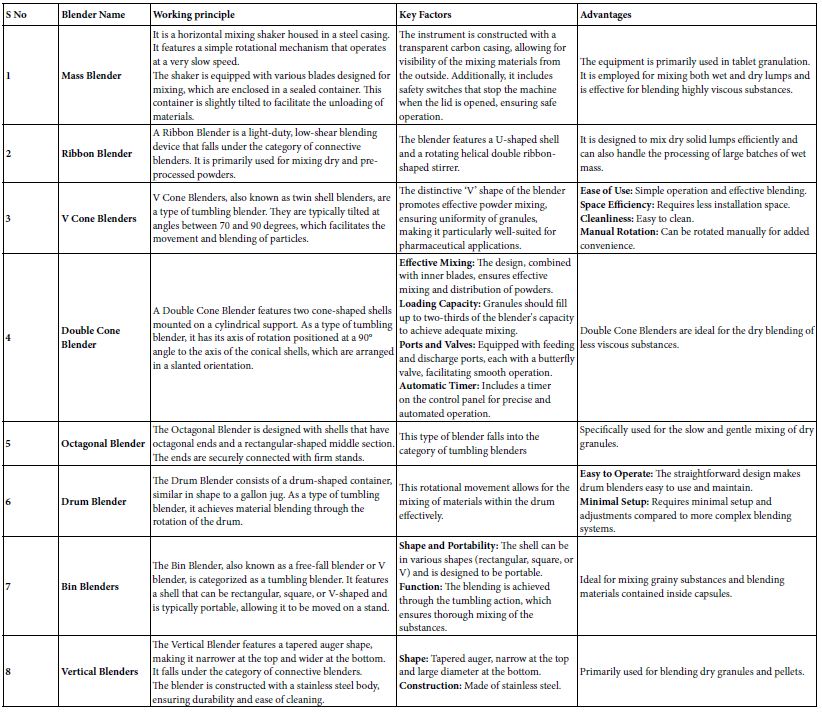
B) Granulation in Pharmaceutical Manufacturing
Granulation is a crucial process in the pharmaceutical industry, particularly in the manufacture of solid dosage forms like tablets and capsules. It involves the formation of granules from a mixture of powders, which can improve the properties of the final product. Purpose of Granulation is to improve flow properties, enhance compressibility, reduce dust and improve uniformity [17,18]. Currently pharmaceutical industry adapted different types of granulation methods such as
i). Dry Granulation
Involves compressing powders into slugs or sheets and then milling them into granules. This method is used when the API is sensitive to moisture or heat. The process includes roller compaction and slugging. Typically roller compactors are used in dry granulation process.
ii). Wet Granulation
Involves adding a liquid binder to the powder mixture, which forms a wet mass that is then dried and sized into granules. This method typically includes preparation of binder solution, granulation, drying and sizing. Typically high shear rapid mixer granulators are used for wet granulation.
iii). Semi-Wet Granulation
A combination of wet and dry process involves in this granulation techniques, where a small amount of liquid binder is used, and the granules are only partially dried. Typically low shear fluid bed granulators are used in semi-wet granulation process.
i). Dry Granulation – Scale-Up Consideration and Industry Perspectives
Dry granulation is an alternative to both direct compression and wet granulation, particularly suited for active pharmaceutical ingredients (APIs) that are sensitive to moisture, have poor flow properties, or possess other physicochemical characteristics that are incompatible with direct compression or wet granulation. Unlike wet granulation, dry granulation does not involve the use of solvents or additional heating, which can introduce challenges related to physical or chemical stability, especially in formulations with amorphous solid dispersions or those prone to chemical degradation. Dry granulation offers several advantages over wet granulation, including a simpler process that is particularly beneficial for APIs that are sensitive to heat or water [19]. The two most commonly used methods for dry granulation are roller compaction and slugging.
Roller Compaction (RC) is a dry granulation technique that simultaneously densifies and agglomerates the powder blend to achieve increased packing density and granule size. In this process, the blend is compacted into ribbons using rollers, which are then milled into granules. Roller compaction reduces the risk of segregation, minimizes dust formation, and produces ribbons that can be processed into granules with improved flow properties. These granules are suitable for various subsequent processes, such as sachet filling, capsule filling, or tableting. Different scales and schematic representation of the roller compaction process is depicted in the Figure 2.
Figure 2: Different types of Roller compacters a) Lab scale model b) Pilot/Commercial Scale c) Schematic presentation of Roller Compactor.
Scaling up of roller compaction involves utilizing traditional large- scale experimental designs to optimize the dry granulation process. This approach can be time-consuming and resource-intensive. To streamline scale-up and minimize the number of experiments, it is crucial to have a deep understanding of the process parameters and the attributes of both the ribbons and granules produced [20]. Key process parameters for roller compaction include roll gap, roll pressure, feed screw speed, roll speed, and roller shape. These parameters must be carefully adjusted to achieve the desired granulation outcomes [21]. The quality of the ribbon, which is the primary product of the roller compaction process, is assessed based on several attributes like Ribbon Density (an indicator of how compacted ribbon is), Ribbon Strength (reflects the mechanical strength of the ribbon), Ribbon Thickness (Affects the granule size and uniformity), Young’s Modulus (Measures the ribbon’s elasticity and rigidity), Ribbon Shape (Impacts the subsequent granule formation), and Moisture Content (Ensures the ribbon’s stability and suitability for further processing). By focusing on these parameters and attributes, it is possible to optimize the roller compaction process effectively while reducing the need for extensive experimentation. The rolling theory for granular solids developed by Johanson describes the pressure distribution along the rolls considering the physical characteristics of the powder and the equipment geometry. The dimensionless number frequently used in roller compaction is determined based on the Johanson theory [22]. Johanson proposed a model that predicts the density of ribbons made by roller compaction using the nip area and the volume between the roll gaps. Johanson proposed distinguishing two regions between the rolls, (i) a slip region where the roll speed is faster than the powder and there is only rearrangement of the particles and (ii) a nonslip region where the powder gets trapped between the rolls and becomes increasingly compacted until the gap. The transition from slip to nonslip region is defined by the so-called nip angle. In the nonslip region, it is assumed that the powder behaves as a solid body being deformed as the distance between the rolls narrows down to the gap. It is further assumed that the deformation has only one axial component such that it can be idealized as uniaxial compression. One source of discrepancy between the predictions of Johanson’s and Reynolds’ model and the ribbon density measurements is the different compaction behaviour of the powder in the roller compactor compared to uniaxial compression tests [23,24] demonstrated that the roller compactor and a compaction simulator lead to different ribbon densities and built a model to account for that difference.
Rowe et al. extended Johanson’s model and proposed a modified Bingham number (Bm*) that represented the ratio of yield point to yield stress as follows:
Where Cs is the screw speed constant, 0 is pre consolidation factor, ρtrue is true density, is circumference of the roll circle, D is the roll diameter, W is roll width, SA roll is roll surface area, S is roll gap, NS is feed screw speed and NR is roll speed. Bm* is easy to determine because the input parameters of Bm*consist of those that can be generally measured in the compaction process. The model- predicted values and the actual test results from WP 120 Pharma and WP 200 Pharma (Alexander werk, Remscheid, Germany) models are shown. By maintaining Bm*, it was possible to obtain a consistent ribbon density between the two operating scales. It was suggested that Bm* can be effectively used for the development of roller compaction scale-up [25]. Case studies suggest that dimensionless numbers for the prediction of ribbon density in dry granulation processes can be used successfully during the Scale-up process (Table 2).
Table 2: Critical process parameters, quality attributes, scale up considerations and industry practices for Roller compaction unit operation.
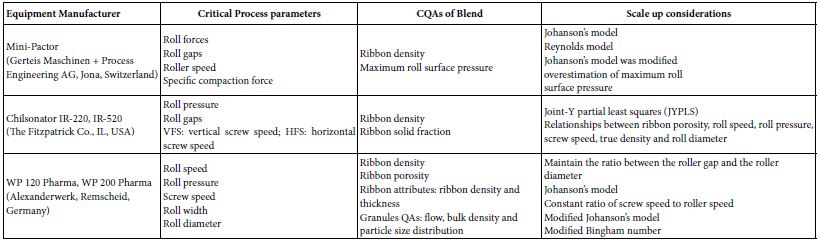
ii). Wet Granulation – Scale-Up Consideration and Industry Perspectives
Wet granulation is a key process in pharmaceutical manufacturing used to produce granules from powders by incorporating a liquid binder (Figure 3).
Figure 3: Different types of wet granulation process equipments used in pharmaceutical development a) Lab model rapid mixer granulator b) Pilot/Commercial scale rapid mixer granulator.
This process is crucial for ensuring that the final granules exhibit desirable properties such as uniformity, good flowability, and compressibility [26]. The choice of equipment for wet granulation includes high-shear Rapid mixer granulators (RMG) and low shear fluid bed granulators (FBG). RMG involves mixing powders with the binder in a high-shear environment. The impeller and chopper facilitate the formation of granules by applying mechanical forces. FBG involves spraying the binder solution onto the powder bed in a fluidized state. The fluidized bed aids in the uniform distribution of the binder and granule formation. Granulation Process involves the dry powders, including the active pharmaceutical ingredient (API) and excipients (e.g., fillers, disintegrants, lubricants), are blended to ensure a uniform distribution. The blended powders are loaded into the high-shear granulator’s mixing bowl. The liquid binder (e.g., water, ethanol, or polymer solution) is sprayed onto the powder bed. This binder helps in forming granules by adhering powder particles together. The impeller rotates on a horizontal plane, creating a high-shear environment that facilitates mixing and initial granule formation. The chopper, rotating either vertically or horizontally, breaks up large lumps and ensures the uniform size of granules by cutting and mixing [27]. Granulation end point determined by the granules continue to grow as the binder is added until they reach the desired size and consistency. The process is typically monitored to ensure that granules are not over granulated or under-granulated. The process is carefully controlled by adjusting parameters such as binder addition rate, impeller speed, and chopper speed. A predefined endpoint, based on granule size or moisture content, is set to determine when the granulation is complete. Scaling up a Rapid Mixer Granulator (RMG) involves translating process parameters from a smaller, laboratory-scale unit to a larger, production-scale unit while maintaining the desired granule quality and consistency (Table 3a and 3b). This process requires careful consideration of equipment design, power requirements, and process parameters. Below tabulated are the guide to some common scale-up calculations for RMG.
Table 3a: Critical process parameters, quality attributes, scale up considerations for RMG granulation unit operation.

Table 3b: Scale up considerations and industry practices for RMG granulation unit operation.
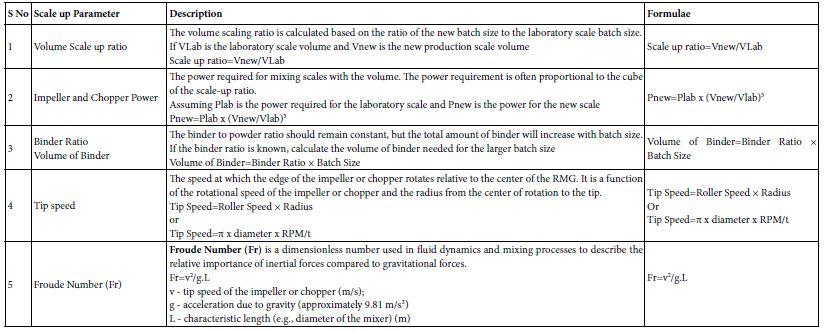
iii). Semi-Wet Granulation – Scale-Up Consideration and Industry Perspectives
Fluid Bed Processor (FBP) for granulation operates by passing hot air at high pressure through a distribution plate located at the bottom of the container, creating a fluidized bed of solid particles. This fluidized state, where particles are suspended in the air, facilitates drying. Granulating liquid or coating solutions are sprayed onto these fluidized particles through a spray nozzle, followed by drying with hot air. The fluidized bed processor operates on the principle of fluidization, where a gas (typically air) is passed through a bed of solid particles at a velocity sufficient to suspend the particles in the gas stream. Air is introduced through a perforated plate or distributor at the bottom of the bed, and as it flows upwards, it lifts the particles, making them behave like a fluid. During fluidization, various processes can be carried out: a binder solution or melt is sprayed onto the particles, causing them to agglomerate; hot air removes moisture from the particles; and a coating solution is applied, which is then dried. The air, now carrying moisture or coating material, exits through the top of the bed. Scaling up a FBP in the pharmaceutical industry involves several calculations and considerations to ensure that the process can be effectively transitioned from a laboratory or pilot scale to full- scale production [28,29]. The process must maintain product quality, efficiency, and compliance with regulatory standards. Here’s a detailed guide on scale-up calculations and key factors for Fluidized Bed Processors. Scaling up a FBP involves maintaining similar fluidization conditions and process outcomes as in smaller scales. Key principles include maintaining the same fluidization regime, similar granulation or coating characteristics, and ensuring that drying or granulation efficiency scales proportionally (Table 4).
Table 4: Scale up considerations and industry practices for FBP granulation.
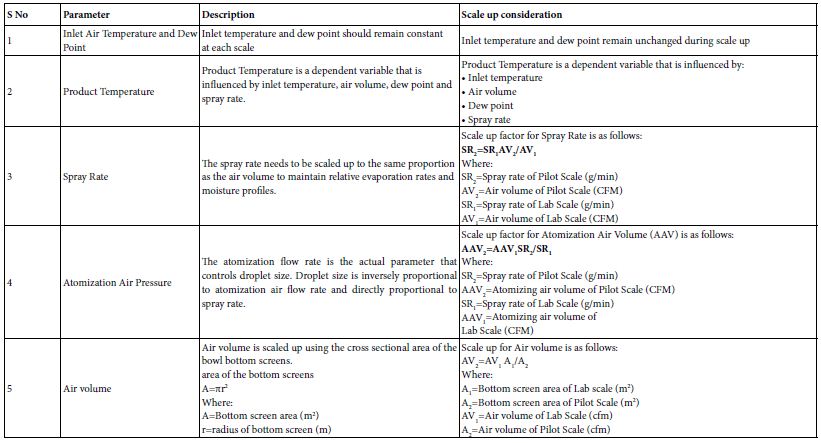
C) Compression in Pharmaceutical Manufacturing
Tablet compression is a critical process in pharmaceutical manufacturing that involves transforming powdered or granulated substances into solid tablets (Figure 4).
Figure 4: Different types of compression machines used in pharmaceutical development a) Lab model Single Punch Tablet Press and b) Pilot/commercial Scale Single Rotary Tablet Press.
Compression is a critical and challenging step in tablet manufacturing. The way a powder blend is compressed directly impacts tablet hardness and friability, which are crucial for dosage form integrity and bioavailability. While the tablet press is essential for the compression process, the preparation of the powder blend is equally important to ensure it is suitable for compression. Understanding the physics and principles of the compression process is vital for managing these operations effectively. For high-dose or poorly compressible drugs, the study of compression becomes particularly important, especially when the relationship between compression force and tablet tensile strength is non-linear. A thorough grasp of compression dynamics also helps resolve many tableting issues, which often stem from various compression-related factors [30,31].
Compression Cycle
Understanding the different stages of the compression cycle is essential for comprehending how powder materials are compacted into tablets. It also provides valuable insights into the various formulation and compression variables that impact the quality of the finished tablet. Compression cycle is divided into following 4 phases: Pre-compression, Main-compression, Decompression and Ejection.
Pre-compression
As the name implies, pre-compression is the initial stage where a small force is applied to the powder bed to create partial compacts before the main compression. This is typically achieved using a pre- compression roller that is smaller than the main compression roller. However, the size of the pre-compression roller and the level of pre- compression force can vary based on the properties of the material being compressed. For instance, powders that are prone to brittle fracture may require a higher pre-compression force compared to the main compression force to achieve increased tablet hardness. In contrast, elastic powders need a gradual application of force to minimize elastic recovery and allow for stress relaxation. Optimal tablet formation is often achieved when the sizes of the main and pre- compression rollers and the forces applied are similar.
Main Compression
During the main compression phase, inter particulate bonds are formed through particle rearrangement, which is followed by particle fragmentation and/or deformation. For powders with viscoelastic properties, special attention to compression conditions is necessary, as these conditions significantly influence the material’s compression behavior and the overall tableting process.
Decompression
After the compression phase, the tablet experiences elastic recovery, which introduces various stresses. If these stresses exceed the tablet’s ability to withstand them, structural failures can occur. For instance, high rates and degrees of elastic recovery may lead to issues such as tablet capping or lamination. Brittle fractures can also occur if the tablet undergoes brittle fracture during decompression. To alleviate stress, plastic deformation, which is time-dependent, can occur. The rate of decompression also influences the potential for structural failure. Therefore, incorporating plastically deforming agents, such as PVP or MCC, is recommended to enhance the tablet’s ability to handle these stresses.
Ejection
Ejection is the final stage of the compression cycle, involving the separation of the tablet from the die wall. During this phase, friction and shear forces between the tablet and the die wall generate heat, which can lead to further bond formation. To minimize issues such as capping or laminating, lubrication is often used, as it reduces ejection forces. Powders with smaller particle sizes typically require higher ejection forces to effectively remove the tablets from the die. Industry perspective is to overall understanding the theoretical aspects of compression helps in selecting the optimal compression conditions for a given tablet product and at the same time can avoid the potential tableting problems thus saving significant time and resources.
D) Wurster Coating in Pharmaceutical Manufacturing
The Wurster fluid bed coating technique is renowned for its versatility and efficiency in coating applications [32]. This method is distinguished by its rapid heat and mass transfer capabilities and its ability to maintain temperature uniformity. Unlike traditional fluidized bed coating, which uses a more straightforward approach, the Wurster method employs a nozzle located at the bottom of a cylindrical draft tube to spray the coating solution. Particles are circulated through this tube, periodically passing through the spraying zone where they encounter fine droplets of the coating solution. This circulation not only ensures thorough mixing but also provides precise control over particle movement and coating quality. Wurster Coating Process is extensively utilized in the pharmaceutical industry for coating powders and pellets. Wurster systems can handle batch sizes ranging from 100 grams to 800 kilograms. This process is ideal for coating particles as small as 100 µm up to tablets. The Wurster coating chamber is typically slightly conical and features a cylindrical partition about half the diameter of the chamber’s bottom. At the base of the chamber, an Air Distribution Plate (ADP), also known as an orifice plate, is installed. The ADP is divided into two areas: the open region beneath the Wurster column, which allows for greater air volume and velocity, and the more restricted areas. As air flows upward through the ADP, particles move past a spray nozzle positioned centrally within the up- bed region of the ADP. This nozzle, which is a binary type, has two ports: one for the coating liquid and one for atomized air. The nozzle creates a solid cone spray pattern with a spray angle of approximately 30-50°, which defines the coating zone. The region outside the cylindrical partition is referred to as the down-bed area. The choice of ADP is based on the size and density of the material being coated. The height of the column regulates the horizontal flow rate of the substrate into the coating zone. As the coating process progresses and the mass of the material increases, the column height is adjusted to maintain the desired pellet flow rate.
Scaling up the Wurster coating process involves increasing the equipment size to handle larger batch capacities, ranging from small lab-scale units to industrial-scale machines (Figure 5).
Figure 5: Different types of Wurster coating equipments used in pharmaceutical development a) Lab model b) Pilot/Commercial scale model.
Larger systems require careful design to maintain consistent coating quality and process efficiency. Equipment dimensions, including the height and diameter of the coating chamber and the size of the Air Distribution Plate (ADP), must be scaled proportionally to ensure effective particle fluidization and coating (Table 5).
Table 5: Scale up considerations and industry practices for Wurster coating.
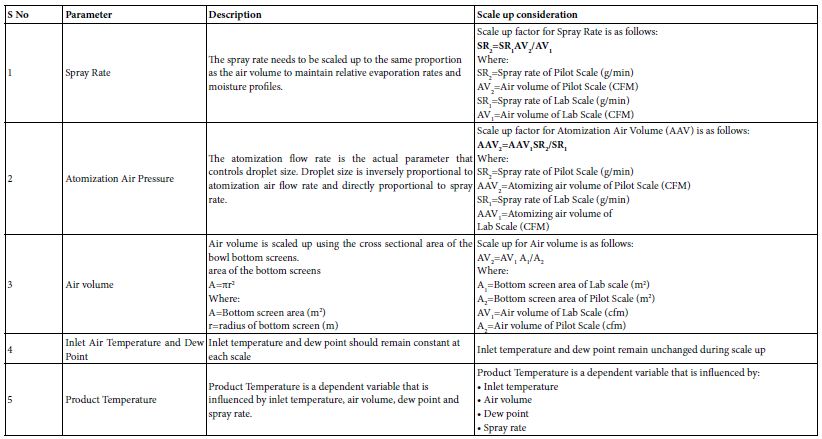
As batch size increases, maintaining optimal airflow dynamics becomes crucial. The airflow rate, velocity, and distribution must be adjusted to ensure uniform coating. Larger systems may require modifications to the ADP to accommodate increased air volume and maintain desired particle circulation and spray pattern. The configuration of spray nozzles needs to be scaled to match the increased batch size. Ensuring consistent liquid atomization and spray pattern is essential to achieve uniform coating thickness. In larger systems, multiple nozzles may be used to cover the expanded coating zone. Process parameters such as temperature, airflow, and coating solution viscosity must be carefully calibrated. Industry perspectives as scale- up introduces more variables, precise control of these parameters is necessary to maintain coating uniformity and avoid issues such as over or under coating. Scaling up involves adjustments in material handling to accommodate the larger volume and ensure smooth transfer and processing of the particles. This includes considerations for feeding systems, particle flow control, and uniform distribution within the coating chamber.
E) Film Coating in Pharmaceutical Manufacturing
Film coating is a widely used technique in pharmaceutical manufacturing to apply a thin layer of coating material onto tablets, and other dosage forms (Figure 6).
Figure 6: Different types of Film coating equipment used in pharmaceutical development a) Lab model and b) Pilot/commercial Scale film coating equipment.
This coating process enhances the appearance, improves the stability, and controls the release of active ingredients in pharmaceutical products. Different film coating formulations can be used to achieve controlled or modified-release properties. This allows for the gradual release of the drug over time, improving therapeutic outcomes and patient compliance. Film coatings can improve the appearance of dosage forms, making them more appealing to patients. Additionally, they can mask the taste of unpleasant drugs, making oral administration more acceptable [33]. Choosing the wrong film coating equipment or using subpar technology can lead to significant film coating defects. These defects can greatly affect the quality, efficacy, and appearance of pharmaceutical products. It’s essential to identify and address these issues to maintain product integrity and ensure compliance. Below is an overview of common film coating defects and their potential causes, as detailed in Table 6a. Scaling up of film coating processes in pharmaceutical manufacturing involves several important considerations to ensure that the coating process remains effective and consistent as production volumes increase Table 6b.
Table 6a: Pharmaceutical film coating defects, route cause and remedial action.
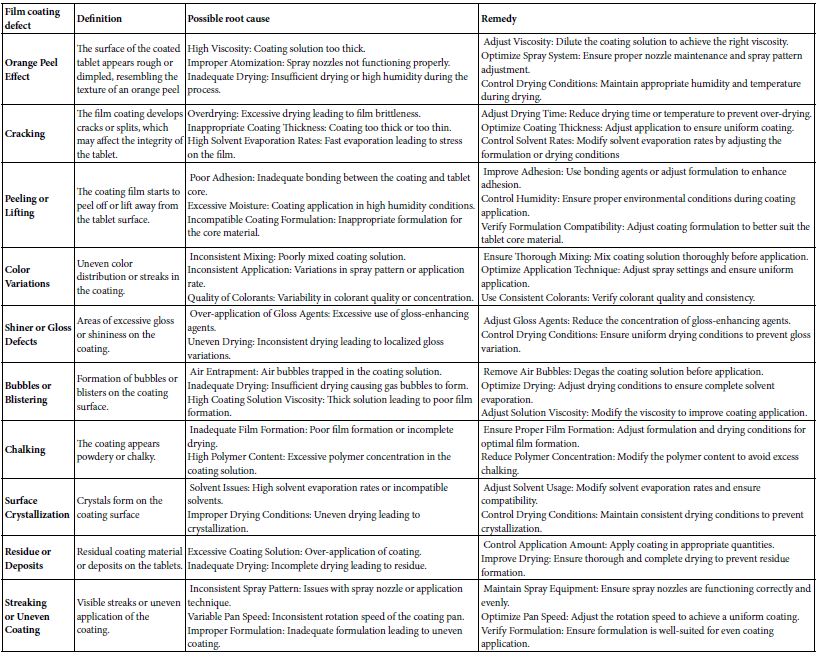
Table 6b: Scale up considerations and industry practices for Film coating.
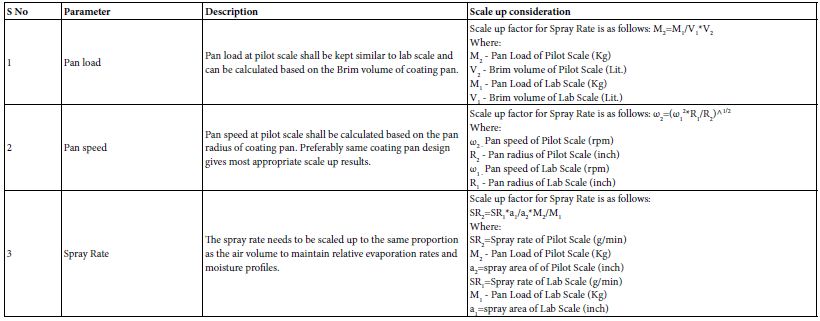

Current Industry Persepctives
Current industry perspectives on scale-up calculations emphasize a comprehensive understanding of both the scientific and operational aspects of production. By leveraging the scale up calculations, advanced methodologies such as Design of Expert (DoE) and quality by design (QbD), along with a keen focus on cost, equipment selection, and regulatory compliance, pharmaceutical companies can navigate the complexities of scaling up oral solid dosage forms effectively. Adapting to technological advancements and maintaining a proactive approach to risk management will be crucial for success in an increasingly competitive landscape.
Conclusion
The scale-up of oral solid dosage forms (OSDFs) is a critical phase in pharmaceutical development that directly influences product quality, regulatory compliance, and market success. The successful scale-up of OSDFs is a multifaceted challenge that requires strategic planning and execution. By focusing on these critical factors integrated processes, quality assurance, economic considerations, regulatory compliance, technological advancements, risk management, and continuous improvement pharmaceutical industries can enhance their chances of delivering high-quality products to the market. As the industry evolves, maintaining a forward-thinking approach will be essential for navigating complexities and ensuring sustainable success in a competitive landscape.
Conflicts of Interest
The authors declare no conflict of interest
Acknowledgement
Authors acknowledge Dr. Sudhakar Vidiyala, Managing Director, Ascent Pharmaceuticals Inc. for his support and encouragement in writing this review article.
References
- Eun HJ, Yun SP, Min-Soo K, Hyung DC (2020) Model-Based Scale-up Methodologies for Pharmaceutical Granulation. Pharmaceutics 12. [crossref]
- Doodipala N, Palem CR, Reddy S, Madhusudan RY (2011) Pharmaceutical development and clinical pharmacokinetic evaluation of gastroretentive floating matrix tablets of levofloxacin. Int J Pharm Sci Nanotech 4: 1461-1467.
- Raval N, Tambe V, Maheshwari R, Pran KD, Rakesh KT (2018) Scale-Up Studies in Pharmaceutical Products Development. In Dosage Form Design Considerations; Academic Press: Cambridge, MA, USA.
- Amirkia V, Heinrich M (2015) Natural products and drug discovery: A survey of stakeholders in industry and academia. Frontiers in Pharmacology 6. [crossref]
- Morten A, Rene H, Per H (2016) Roller compaction scale-up using roll width as scale factor and laser-based determined ribbon porosity as critical material attribute. Eur J Pharm Sci 87: 69-78. [crossref]
- Mazor A, Orefice L, Michrafy A, Alain DR, Khinast JG (2018) A combined DEM & FEM approach for modelling roll compaction process. Powder Technol 337: 3-16.
- Vladisavljevi´c GT, Khalid N, Neves M A, Kuroiwa T, Nakajima M, et al. (2013) Industrial lab-on-a-chip: Design, applications and scale-up for drug discovery and delivery. Adv. Drug Deliv Rev 65(11-12): 1626-1663. [crossref]
- Palem CR, Gannu R, Yamsani SK, Yamsani VV, Yamsani MR (2011) Development of bioadhesive buccal tablets for felodipine and pioglitazone in combined dosage form: in vitro, ex vivo, and in vivo characterization. Drug delivery; 18(5): 344-352. [crossref]
- U.S: Food and Drug Administration. Pharmaceutical cGMPS for the 21st Century – A Risk-Based Approach: Second Progress Report and Implementation Plan. FDA website. Drugs section 2003.
- Palem CR, Gannu R, Doodipala N, Yamsani VV, Yamsani MR (2011) Transmucosal delivery of domperidone from bilayered buccal patches: in Vitro, ex Vivo and In Vivo characterization. Archives of pharmacal research 34: 1701-1710. [crossref]
- Mahdi Y, Mouhi L, Guemras N, Daoud K (2016) Coupling the image analysis and the artificial neural networks to predict a mixing time of a pharmaceutical powder. J Fundam Appl Sci 8: 655–670.
- Moakher M, Shinbrot T, Muzzio FJ (2000) Experimentally validated computations of flow, mixing and segregation of non-cohesive grains in 3d tumbling blenders. Powder Technol 109: 58-71.
- Cleary PW, Sinnott MD (2008) Assessing mixing characteristics of particle-mixing and granulation devices. Particuology 6: 419-444.
- Mendez ASL, Carli de G, Garcia CV (2010) Evaluation of powder mixing operation during batch production: Application to operational qualification procedure in the pharmaceutical industry. Powder Technology 198: 310–313.
- Arratia P.E, Duong N h, Muzzio F.J, Godbole P, Lange A, et al. (2006) Characterizing mixing and lubrication in the bohle bin blender. Powder Technol 161: 202–208.
- Adam S, Suzzi D, Radeke C, Khinast JG (2011) An integrated quality by design (QbD) approach towards design space definition of a blending unit operation by discrete element method (DEM) simulation. Eur J Pharm Sci 42: 106-115. [crossref]
- Palem CR, Dudhipala N, Battu SK, Goda S, Repka MA, et al. (2015) Combined dosage form of pioglitazone and felodipine as mucoadhesive pellets via hot melt extrusion for improved buccal delivery with application of quality by design approach. Journal of Drug Delivery Science and Technology; 30: 209-219.
- Palem CR, Gannu R, Yamsani SK, Yamsani VV, Yamsani MR (2011) Development of bioadhesive buccal tablets for felodipine and pioglitazone in combined dosage form: in vitro, ex vivo, and in vivo characterization. Drug delivery 18: 344-352. [crossref]
- Reddy PC, Dudhipala NR, Goda S, Pokharkar PB (2016) Development and optimization of atorvastatin calcium-cyclodextrin inclusion complexed orally- disintegrating tablets with enhanced pharmacokinetic and pharmacodynamic profile. Int J of Pharm Sci and Nanotechnology (IJPSN) 9: 1-11. [crossref]
- Teng Y, Qiu Z, Wen H (2009) Systematic approach of formulation and process development using roller compaction. Eur J Pharm Biopharm 73: 219-229. [crossref]
- Kleinebudde P (2004) Roll compaction/dry granulation: Pharmaceutical applications. Eur J Pharm Biopharm 58: 317-326.
- Gago AP, Reynolds G, Kleinebudde P (2018) Impact of roll compactor scale on ribbon density. Powder Technol 337: 92-103.
- Johanson J (1965) A rolling theory for granular solids. J Appl Mech 32(4): 842-848.
- Reynolds G, Ingale R, Roberts R, Kothari S, Gururajan B (2010) Practical application of roller compaction process modeling. Comput Chem Eng 34: 1049–1057.
- Reimer HL, Kleinebudde P (2018) Hybrid modelling of roll compaction processes with the Styl’One Evolution. Powder Technol 341: 66–74.
- Rowe JM, Crison JR, Carragher TJ, Vatsaraj N, Mccann RJ, et al. (2013) Mechanistic Insights into the Scale-Up of the Roller Compaction Process: A Practical and Dimensionless Approach. J Pharm Sci 102: 3586-3595. [crossref]
- Palem CR, Kumar BS, Gannu R, Yamsani VV, Repka MA (2012) Role of cyclodextrin complexation in felodipine-sustained release matrix tablets intended for oral transmucosal delivery: In vitro and ex vivo characterization. Pharmaceutical Development and Technology 17: 321-332. [crossref]
- Rambali B, Baert L, Massart D L (2003) Scaling up of the fluidized bed granulation process. Int J Pharm 252: 197-206. [crossref]
- Victor EN, Ivonne K, Maus M, Andrea S Daniela S (2021) A linear scale-up approach to fluid bed granulation. Int J Pharm 598: 120-209. [crossref]
- Patel S, Kaushal AM, Bansal AK (2006) Compression Physics in the Formulation Development of Tablets. Critical Reviews TM in Therapeutic Drug Carrier Systems 23: 1-65. [crossref]
- Mohan S (2012) Compression Physics of Pharmaceutical Powders: A Review. Int J of Pharm Sci and Research 3: 1580-1592. [crossref]
- Teunou E, Poncelet D (2002) Batch and continuous fluid bed coating – review and state of the art. Journal of Food Engineering.
- Ahmad S (2022) Pharmaceutical Coating and Its Different Approaches, a Review. Polymers 14. [crossref]