DOI: 10.31038/IDT.2024522
Abstract
The top five candidate DNA aptamer sequences developed and published previously by Bruno et al. (BMC Research Notes 5: 633, 2012) against Crimean- Congo Hemorrhagic Fever virus envelope glycoprotein precursor peptides were screened for plaque reduction activity against viable CCHF Oman virus in a BSL-4 laboratory. Statistically significant reductions in plaque forming unit (pfu) counts for CCHF using SW-13 cell cultures were obtained when pretreated with these aptamer DNA sequences which demonstrated much greater efficacy versus a polyadenine 49mer control oligonucleotide at the same concentrations.
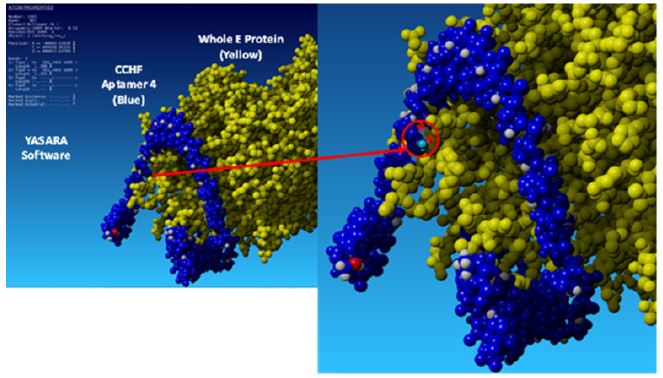
Keywords
Aptamer, Crimean-Congo hemorrhagic fever, Glycoprotein, Plaque, Virus
Introduction
Passive immunity for lethal hemorrhagic viruses such as Ebola has been demonstrated using convalescent antiserum [1-4]. Similarly, humanized monoclonal antibodies produced by Eli Lilly and Regeneron proved effective as passive immunity agents to protect patients suffering severe COVID during the pandemic [5].
Unfortunately, convalescent human antisera and humanized monoclonal antibodies are difficult and rather expensive to develop and mass produce. Nucleic acid aptamers present a much less expensive and more facile development alternative to humanized antibodies for industrial scale up of biologics to bind and block or inhibit progression of deadly viruses. A number of successful in vitro experiments exist reporting reductions in the number plaque forming units (pfu) or blockage of infections when viruses are pretreated with specific cognate aptamers and then added to cultures of mammalian host cells [6-9].
Crimean-Congo Hemorrhagic Fever (CCHF) virus is a widespread tick-borne virus member of the Nairoviridae with mortality reported as high as 30% in affected humans primarily across Europe and Africa [10]. As such, this virus is the subject of intense research [10-13]. While small molecule viral inhibitors have demonstrated significant efficacy against CCHF [12], humanized monoclonal antibodies are a prime target for the development of therapeutic medical countermeasures as well [10,13]. Human antibodies are known to synergistically neutralize the virus when bound to the envelope glycoprotein precursor [10]. In this work, one of the top 5 aptamers was shown to cause up to a 92.2% reduction in plaque forming units (pfu) and the other four aptamer DNA sequences also appear to exhibit significant efficacy as passive immunity pretreatment agents at least thus far in vitro.
Materials and Methods
Aptamer Development and DNA Sequencing
The SELEX aptamer development process against synthetic peptides derived from the CCHF envelope glycoprotein precursor (GenBank AHL45281.1) attached to magnetic microbeads was previously described by Bruno et al. [14]. All DNA aptamers or control oligonucleotides were synthesized by Integrated DNA Technologies (Coralville, IA).
SW-13 Plaque Reduction Assay
The traditional pfu reduction assay did not work well with Vero cells in initial experiments for unknown reasons. Therefore, the method of Paragas et al. [11] was used with human SW-13 adrenal cortex epithelial cells (ATCC CCL-105) except that crystal violet dye was used instead of neutral red. SW-13 cells were cultured in the BSL- 4 4 laboratory of the Texas Biomedical Research Institute (TBRI; San Antonio, TX, USA) to a confluent monolayer in Dulbecco’s minimum essential medium (DMEM) plus 10% fetal calf serum (FCS) in 6-well plates at 37˚C with 5% CO2. The culture medium was replaced with a 1:2,500 dilution of stock CCHF Oman strain in 0.5 ml of DMEM plus 2% FCS with or without the aptamer levels indicated in the figures (0 to 1,900 nanomoles of aptamers) for 60 min at 37˚C with gentle rocking. Culture medium was removed from the wells and 5 ml of DMEM plus 1% penicillin-streptomycin-L-glutamine mixture and 16% methylcellulose overlay was added to each well. Thereafter, 6-well plates were incubated in a humidified incubator at 37° with 5% CO2 for 7-8 days. At the end of this week-long incubation period, the DMEM and methylcellulose overlays were removed and 5 ml of room temperature 10% neutral buffered formalin was added to each well as a fixative. Plates were refrigerated between 2°C to 8°C overnight (12 h minimum). The following day, the formalin was removed and wells were washed with Phosphate Buffered Saline (PBS). Plaque counts were performed manually by two technicians in agreement about the numbers of pfus following staining of each well in 1 ml of crystal violet, followed by removal of the dye, a gentle rinse with fresh tap water and air drying. Plaque counts were subjected to ANOVA statistical testing and found to show statistically significant differences for all of the aptamer-pretreated groups versus the blanks and ploy-A controls with p values <0.01.
Results
Figure 1 shows the results of initial plaque reduction studies in which the means of triplicate well readings with 2X standard deviation bars appeared to produce a >50% reduction in the mean number of pfu per well from pretreatment of the Oman CCHF virus with 47 nmoles of aptamer 2 versus the >120 pfu for the blank control without any aptamer pretreatment additive. The blank in this initial experiment was comparable in number of pfu versus the poly-A 49mer control wells. The other aptamers in this first experiment did not reduce plaque formation >50%, but also demonstrated statistically significant reductions in plaque formation.
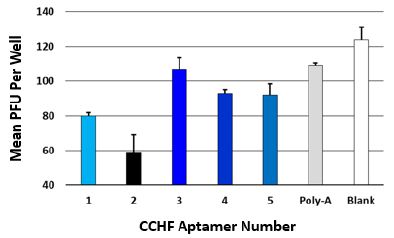
Figure 1: Initial plaque reduction screening experimental results for the top 5 aptamers and a poly-adenine 49mer all at 47 nmole of DNA per well except for the zero aptamer added blank. The averages and 2X standard deviation bars for 3 measurements are shown for each group.
In the second set of higher dose studies (Figure 2) with only aptamers 1 and 2, the aptamers exhibited a >90% reduction (up to 92.2% for aptamer 1 at 1,900 nmoles of aptamer) which was dose- dependent. Although the poly-A control exhibited some dose- dependent reduction in plaque formation as well in Figure 2, it was not nearly as effective at plaque reduction versus the more specific CCHF aptamers (~50% reduction versus >90%) at the 1,900 nmole concentration level.
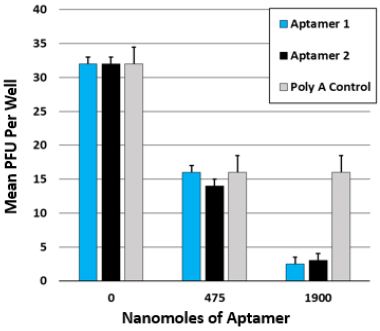
Figure 2: Dose-dependence plaque reduction results for aptamers 1 and 2 versus the poly-A 49mer and the blank group at the higher DNA concentrations indicated. The averages and 2X standard deviation bars for 3 measurements are shown for each group.
Discussion
Jalali et al. developed numerous aptamers against the conserved internal nucleoprotein (NP) of CCHF which produced excellent reagents for sensitive detection [15] of many different variants of lysed CCHF viruses. However, anti-NP aptamers are not very useful as therapeutics or prophylactics for passive immunity since they do not bind the viral surface or prevent viral fusion with the host cell [6]. Here the author describes the first in vitro testing of aptamers developed against the CCHF envelope glycoprotein precursor complex [14] which could inhibit or prevent CCHF viral fusion and host cell entry as demonstrated by the present data (Figures 1 and 2). The top 5 aptamer DNA sequences reported here (Table 1) were mostly generated against synthetic peptides in the Gc region of the CCHF envelope glycoprotein precursor [14] (amino acids 1041 through 1684) which form spikes on the viral surface involved in viral host cell entry [16]. The author has previously reported 3-D molecular models of the top 5 aptamers docked with the CCHF envelope glycoprotein precursor [17] which bear some resemblance to the precursor domain II regions bound by the antibodies Mishra et al. have reported [10] making the results reported here quite plausible.
Table 1: Aptamer DNA sequences.
Aptamer No. |
DNA Sequence 5’-3’ |
1 |
ACA GTT AGA GCT TGC CGT ATG CCT TTG TTA ACA TAA |
2 |
ACT AAC CGA ATG GCA GTT TCC CCC TTA TCC ATC TAT |
3 |
GGG ATA GGG TCT CGT GCT AGA TG |
4 |
CGC TGA AGC AAG ACA TTA TCG GGA CAT TGC CGT GA |
5 |
TGA CAC GCG TAC GGG TCC GGA CAT GTC ATA ACG GAC |
Conclusions
In this preliminary in vitro study, the top 5 aptamer candidates from Bruno et al.’s previous publication [14] demonstrated solid in vitro potential to act as passive immunity biologics. CCHF aptamers 1 and 2 in particular demonstrated strong dose dependence in vitro with >90% efficacy at the 1,900 nmole dose and deserve further research attention as possible alternatives to antibodies for the passive therapy of CCHF. The biggest issues with using aptamers in vivo is their small size making them subject to rapid kidney clearance and susceptibility to serum nucleases. However, aptamer pharmacokinetics can be greatly enhanced by covalent addition of heavier inert blocking agents such as polyethylene glycol or some proteins to the 3’ end [18-20].
Acknowledgments
Funding was provided by a U.S. Defense Department SBIR Contract No. W911SR22P0007. The author thanks Dr. Ricardo Carrion and Dr. Michal Gazi of the Texas Biomedical Research Institute (TBRI) in San Antonio, TX for maintaining the SW13 host cell cultures and conducting plaque assays in the BSL-4 laboratory.
References
- PJ Lachmann (2014) Traditional passive immune therapy for emerging Ebola Emerg Microbes Infect 3: e81. [crossref]
- S Lu (2014) Using convalescent whole blood or plasma as passive immune therapy for the global war against Emerg Microbes Infect 3: e80. [crossref]
- Casadevall, LA Pirofski (2015) The Ebola epidemic crystallizes the potential of passive antibody therapy for infectious PLoS Pathog 11: e1004717. [crossref]
- E Mire, JB Geisbert, KN Agans, EP Thi, AC Lee, et al. (2016) Passive Immunotherapy: Assessment of Convalescent Serum Against Ebola Virus Makona Infection in Nonhuman Primates. J Infect Dis 214: S367-S374. [crossref]
- N Cimolai (2021) Passive Immunity Should and Will Work for COVID-19 for Some Clin Hematol Int 3: 47-68.
- SH Jeon, B Kayhan, T Ben-Yedidia, R Arnon (2004) A DNA aptamer prevents influenza infection by blocking the receptor binding region of the viral hemagglutinin. J Biol Chem 279: 48410-48419. [crossref]
- SCB Gopinath, PKR Kumar Aptamers that bind to the hemagglutinin of the recent pandemic influenza virus H1N1 and efficiently inhibit Acta biomaterialia 9: 8932-8941. [crossref]
- M Wongphatcharachai, P Wang, S Enomoto, RJ Webby, MR Gramer, et al. (2013) Neutralizing DNA aptamers against swine influenza H3N2 J Clin Microbiol 51: 46-54. [crossref]
- M Wang, MC Hao, Y Huangfu, KZ Yang, XQ Zhang, et al. (2024) A Universal Aptamer for Influenza A Viruses: Selection, Recognition, and Infection Inhibition, 7: 249-258.
- K Mishra, J Hellert, N Freitas, P Guardado-Calvo, A Haouz, et (2022) Structural basis of synergistic neutralization of Crimean-Congo hemorrhagic fever virus by human antibodies. Science 375: 104-109. [crossref]
- J Paragas, CA Whitehouse, TP Endy, M Bray (2004) A simple assay for determining antiviral activity against Crimean-Congo hemorrhagic fever Antiviral Res 62: 21-25. [crossref]
- O Ferraris, M Moroso, O Pernet, S Emonet, A Ferrier Rembert, et (2015) Evaluation of Crimean-Congo hemorrhagic fever virus in vitro inhibition by chloroquine and chlorpromazine, two FDA approved molecules. Antiviral Res 118: 75-81. [crossref]
- EJ Mendoza, B Warner, D Safronetz, C Ranadheera (2018) Crimean-Congo hemorrhagic fever virus: Past, present and future insights for animal modelling and medical Zoonoses Public Health 65: 465-480. [crossref]
- JG Bruno, MP Carrillo, AM Richarte, T Phillips, C Andrews, et al. (2012) Development, screening, and analysis of DNA aptamer libraries potentially useful for diagnosis and passive immunity of BMC Res Notes 5: 633. [crossref]
- T Jalali, M Salehi-Vaziri, MH Pouriayevali, SLM Gargari (2021) Aptamer based diagnosis of crimean-congo hemorrhagic fever from clinical Sci Rep 11: 12639. [crossref]
- K Mishra, CL Moyer, DM Abelson, DJ Deer, KEl Omari, et (2020) Structure and Characterization of Crimean-Congo Hemorrhagic Fever Virus GP38. Journal of virology 94: e02005-19. [crossref]
- JG Bruno (2022) Successes and Failures of Static Aptamer-Target 3D Docking Int J Mol Sci 23. [crossref]
- JG Bruno, R Crowell (2008) Selective glutaraldehyde-mediated coupling of proteins to the 3’-adenine terminus of polymerase chain reaction J Biomol Tech 19: 177-183. [crossref]
- H Dougan, DM Lyster, CV Vo, A Stafford, JI Weitz, et al. (2000) Extending the lifetime of anticoagulant oligodeoxynucleotide aptamers in blood. Nucl Med Biol 27: 289-297.
- PS Keefe AD, Ellington A (2010) Aptamers as therapeutics. Nat Rev Drug Discov 9: 537-550. [crossref]